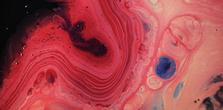
How Sleep Impacts Aging
Sleep is incredibly important for the human body as a whole as well as on the cellular level. What causes alterations in the quantity and quality of sleep and what are the consequences of these changes are quite important questions. What changes exacerbate aging and a more dysfunctional physiology and what changes are caused by aging that are detrimental to your health as well. We will cover the characterization of related changes in sleep structure on both the macro and micro scale and cover the differences between men and women when it comes to sleep and age.
sleep
13 mins
By: Daniel Tawfik
How Sleep Impacts Aging
Mander, Bryce A et al. “Sleep and Human Aging.” Neuron vol. 94,1 (2017): 19-36. doi:10.1016/j.neuron.2017.02.004
Sleep is incredibly important for the human body as a whole as well as on the cellular level. What causes alterations in the quantity and quality of sleep and what are the consequences of these changes are quite important questions. What changes exacerbate aging and a more dysfunctional physiology and what changes are caused by aging that are detrimental to your health as well. We will cover the characterization of related changes in sleep structure on both the macro and micro scale and cover the differences between men and women when it comes to sleep and age.
Macro Sleep Changes:
First let's cover alterations in sleep that occur in your body as a whole or macro sleep changes. When you age your quality and quantity of sleep changes, you yourself can attest to this. Once you're into your 50’s your sleep architecture goes through some radical changes, as shown in figure 1. Now these changes are not universal and each individual will present different changes in their sleep architecture, if any at all.
Macro Sleep Changes:
Advanced sleep timing - Sleep occurs between 6 p.m. and 9 p.m. with waking between the hours of 2 a.m. and 5 a.m.
Longer sleep-onset latency - The longer it takes for sleep to occur, the longer it will take to reach the first REM sleep stage.
Shorter overall sleep duration - The sleep that does occur, happens in shorter than usual time spans.
Increased sleep fragmentation - Sleep fragmentation is the total number of awakenings and transition to stage 1 sleep divided by the total sleep time, or those brief moments of wakefulness that occur when you're sleeping.
More fragile sleep - Sleep that is easily disrupted or waking up because of every little bump in the night.
Reduced amount of deeper NREM sleep - The reduction of sleep in which your body begins to relax.
Increased time spent in lighter NREM Stages 1 and 2 - While sleeping you will spend more time in NREM s\Stages 1 and 2
Shorter and fewer NREM-REM sleep cycles - While sleeping, you will only experience a few cycles between non-REM and REM, compared to the standard amount for a healthy human body.
Increased time spent awake throughout the night - Sleep that is constantly disrupted by the classic tossing and turning.
Macro Sleep Changes:
Age-related reduction of time in REM sleep might pose a more distant threat for those in their 80’s due to only emerging then as a stand-alone issue but is often more so linked to changes within your NREM sleep cycles or as symptoms of degenerative dementia. Ohayon et al., 2004, Van Cauter et al., 2000, Brayet et al., 2015, Hita-Yañez et al., 2012, Petit et al., 2004,
Additionally, the increase in naps during the day also increases as you age, with 10% of adults aged 55-64, and 25% of adults aged 75-84, reporting daytime naps. With roughly half of these two groups’ naps being unplanned, which is consistent with the claim that 1 in 4 of older adults report that daytime sleepiness impairs their daytime plans on a regular basis. These daytime abnormalities may reflect the sleep issues that affect older adults were discussed earlier. Foley et al., 2007
Excessive daytime sleepiness and naps are not universal for old age, with some adults reporting diminished or less daytime sleepiness as well. Dijk et al., 2010 The one factor that appears to control whether or not an adult will be prone to daytime sleepiness or naps is the presence of multiple conditions such as chronic pain, depression, sleep disorders, and frequent urination during the night. Foley et al., 2007, Vitiello, 2009. The propensity of daytime sleepiness can be higher in an otherwise healthy older adult than a younger adult in the evening despite the circadian alerting signal being at its peak in young adults. Münch et al., 2005 Thus, the discrepancies between daytime sleepiness in older adults appears to partially depend on the time of day and/or circadian preference of the individual adults being compared. This becomes further relevant given the advancement in circadian preference in older adults, wherein older adults shift to early bedtimes and early wake times. Monk, 2005
Micro Sleep Changes:
In addition to larger macros sleep changes, there are just as significant changes within the signature electrical oscillations of sleep. Signature electrical oscillations, which are measured with electroencephalography (EEG), are most prominent within NREM sleep and two of its fellow oscillations, slow waves and sleep spindles, which are the pattern of brain waves that occur during NREM sleep. (fig 1).
One method of quantifying slow waves is through the measurement of spectral power in the 0.5-4.5 Hz range during NREM sleep or slow wave sleep, also known as slow wave activity (SWA). While reductions in SWA are observed in middle-aged adults and becomes especially pervasive in older adults.Dijk et al., 1989, Landolt and Borbély, 2001, Landolt et al., 1996, Mander et al., 2013.
It's important to note that age-related decreases in SWA are not evenly distributed with respect to head topography or sleep cycles throughout the night. Instead, maximal age-related decrements in absolute SWA are observed over the prefrontal cortex derivations and in the first NREM sleep cycles, with 75%-80% reductions on average relative to you adults (Fig 1). Dijk et al., 1989, Landolt and Borbély, 2001, Landolt et al., 1996, Mander et al., 2013
SWA is tightly bound to the homeostatic drive to sleep following continued wakefulness, that is the longer you're awake, the greater the pressure and desire to sleep is, additionally the amount of SWA will increase proportional to the time spent awake. Borbély, 1982. For young adults, SWA is highest within the first NREM cycle of the night and then SWA decreases as the night progresses in successive NREM sleep cycles. This decrease in SWA reflects the homeostatic dissipation of sleep pressure as you sleep through the night; that is, the more tired you are, the more slow wave activity there will be (Fig 2). Landolt and Borbély, 2001, Landolt et al., 1996
The process of homeostatic sleep regulation, including SWA, is also changed as a function of aging. The exponential slope of SWA dissipation through the night is less in older adults when compared to those younger (Fig 2A). Landolt and Borbély, 2001 Landolt et al., 1996 Additionally, homeostatic increases in slow wave sleep time and SWA in response to staying awake to the point of sleep deprivation or selective slow wave sleep suppression are blunted within older adults compared to younger adults (Fig 2B). Landolt and Borbély, 2001, Munch et al., 2004. This finding has been interpreted as an impairment of the homeostatic regulation of SWA in older adults. This has been interpreted as an impairment in SWA homeostatic regulation in older adults, with the changes in these homeostatic SWA features in aging are also observed in the prefrontal cortex. Dijk et al., 2010, Munch et al., 2004.
Underlying the changes in SWA is the expression of two NREM slow wave features, the amplitude of slow waves and the density of slow waves, being significantly reduced within middle-aged adults. This reduction is further exacerbated as you age, with further reductions in amplitude and density.Carrier et al., 2011, Dubé et al., 2015. Note that these age-related fluctuations in amplitude and density are not uniform across the brain and that these age-related differences are more prevalent during the 1st and 2nd stages of NREM sleep cycles. Additionally the slope of the slow waves becomes increasingly shallower as you age. These changes suggest that aging may diminish and degrade the synchronized neuronal en masse firing that creates the sleep oscillations, via the disruption of the polarization needed to shape the slow waves. Beenhakker and Huguenard, 2009.
Another drastic change is the reduction of slow wave frequency to roughly 0.1 Hz in older adults. This reduction in frequency can also be observed throughout the brain as opposed to the area specific changes of amplitude and density. Surprisingly the reduction of slow wave frequency is not found within older adults with Beta-amyloid (Aβ) burden and adults with poor memory retention. This link possibly give credence to using slow wave frequency as a new sleep biomarker in distinguishing normal aging versus abnormal aging within the context of Alzheimer's disease pathophysiology. Mander et al., 2016a, Carrier et al., 2011.
The sleep spindle, another definer of NREM sleep oscillation, goes through its own changes during your later life. The sleep spindles reflect the transient bursts of oscillatory activity in the 12-15 Hz range and are generated via corticothalamic networks interacting with the reticular nucleus of the thalamus. Unsurprisingly, sleep spindle frequency is reduced in middle-aged and older adults when compared to young adults. This further increases throughout the night, with the largest age-related impairments being observed up to 50% in the final sleep cycles of the night, as opposed to the faster frequency spindles which are more predominant in young adults. Carrier et al., 2011, De Gennaro and Ferrara, 2003, Mander et al., 2014, Martin et al., 2013, Steriade et al., 1987, Dijk et al., 1989, Landolt et al., 1996.
There are three age-related disruptions in sleep spindles that affect older adults. Firstly, we have reduction in spectral power in the frequency range of sleep spindles generated with the number of sleep spindles declines significantly as you age into your later years. Secondly, the unique features of the spindle waveform are similarly affected as we age and this also appears to contribute to the overall reduction in the signal power that's connected to sleep spindles, with a decrease in the duration, peak, and mean amplitude when you compare older adults to younger adults. Now with slow waves you have these changes are temporally specific to different regions of the brain throughout the night (Fig 1B).De Gennaro and Ferrara, 2003, Mander et al., 2014, Martin et al., 2013,
It's also good to note that age-related reductions in sleep spindles can be readily seen even when the sleep stage in which they wake up doesn't have any changes itself. A good examples would be when you have stage 2 NREM sleep duration not changing but the sleep spindles within stage 2 NREM are reduced. Additionally, make note that the characteristic reduction in total NREM sleep is directly related with the selective loss of stages 3 and 4 of NREM sleep. Thus, even when older adults get the same amount of NREM sleep time, important differences in the density and slow waves can be seen. Therefore, the measurement of NREM sleep stage duration alone cannot capture all of the information with regards to the age-related differences in slow wave expression. De Gennaro and Ferrara, 2003, Fogel et al., 2016, 2016b, Martin et al., 2013, Feinberg and Carlson, 1968, Carrier et al., 2011, Dubé et al., 2015.
All these findings provide evidence for a model in which age-related changes in macro-levels sleep architecture can, and are more often than not, mechanistically distinct from the micro-level changes in sleep oscillations.
.png?u=https%3A%2F%2Fimages.ctfassets.net%2Fzvzqa1d1gh0f%2FF7d6V1Awvsj6QNvPA11dT%2Fc33f9f1ce62bca636203006ce130fc25%2Fimage__2_.png&a=w%3D119%26h%3D181%26fit%3Dcrop%26crop%3Dcenter%26fm%3Dpng%26q%3D75&cd=2022-09-29T17%3A25%3A56.272Z)
image (2)
.png?u=https%3A%2F%2Fimages.ctfassets.net%2Fzvzqa1d1gh0f%2FymvvNUdnnk4d0N9Ux2Jji%2F799e4e1d4aa47a7e115894817737d1a5%2Fimage__3_.png&a=w%3D172%26h%3D261%26fit%3Dcrop%26crop%3Dcenter%26fm%3Dpng%26q%3D75&cd=2022-09-29T17%3A26%3A37.425Z)
image (3)
Sleep, Aging, and Gender.
While the differences between younger and older adults with regards to the macro and micro sleep changes is reliably distinct, the degree in which these older adults suffer sleep disruption varies wildly. This large inter-individual variability means that age is not the sole determining factor of sleep disruption in older adults. Other factors that interact with the aging process must then be determining whether older adults will suffer from age-related decline in both sleep quantity and quality.
So we all know that sex has a huge factor on your physiological functions throughout your life. Sex is a key factor with determining levels of sleep disruption with older men suffering from a greater disruption in NREM sleep when compared to women of the same age. A comprehensive study further demonstrates that increasing age is reliably associated with the same detrimental effects previously described: decreased slow wave time, reduced sleep efficiency, increased NREM stage1 sleep time, increased number of waking up, and decreases in REM sleep time (Fig 3). Men over the age of 70 have a 50% reduction in their slow wave sleep when compared to men under the age of 55, while still having an increase in NREM stages 1 and 2. When contrasted against women, there are no significant reductions in slow wave sleep nor increases in NREM sleep times when you compare older and younger women. Now, when you compare men and women, you have men over the age of 70 having more than a 3-fold deficit in slow wave sleep time compared with women of the same age group. Further meta-analyses have replicated these findings of sex-specific differences in slow wave sleep time in older aged adults. While seemingly subject to differences in slow wave sleep time, both men and women showed moderate reductions in REM sleep time, but this suggests that a sex-independent deterioration of this sleep stage. Redline et al., 2004, Ohayon et al., 2004
Sex also causes age-associated changes in slow wave sleep homeostasis, with older men showing significantly less homeostatic slow wave sleep rebound during recovery sleep following sleep deprivation than women of the same age. When you compare basic sleep stages, both older men and women show very similar homeostatic rebounds in REM sleep during post-deprivation nights. Thus sex-dependent and sex-independent effects emerge in older age, which further suggests that some homeostatic mechanisms remain functionally equivalent in both older men and women, like REM sleep, and some are shown to be strongly sex-dependent, like slow wave sleep.Reynolds et al., 1986
Now, when or what age this stratifying sex-dependent effect takes hold is still unclear, with both men and women in their 20’s showing no signs of NREM sleep differences. Although, there is evidence that points to these differences emerging in your mid-30’s with the measurement of SWA. Men in their mid-30’s have been shown to have a divergence which can be seen in the EEG spectral measure of SWA, which is roughly 50% lower in men in their mid-30’s when compared to their 20’s. The same comparison in women is shown to be only about 25% lower in women in their mid-30’s when compared to their 20’s. Further evidence on if and when these gender-dependent changes is sparse and lacking. Ehlers and Kupfer, 1997, Van Cauter et al., 2000
.png?u=https%3A%2F%2Fimages.ctfassets.net%2Fzvzqa1d1gh0f%2F2xm0L2OIaLHHRpU2gJZYHj%2Fd874fb0290e503d86e56324affc0c4ae%2Fimage__4_.png&a=w%3D126%26h%3D217%26fit%3Dcrop%26crop%3Dcenter%26fm%3Dpng%26q%3D75&cd=2022-09-29T17%3A28%3A00.386Z)
image (4)
Sleep is incredibly important, we spend a large portion of our lives sleeping. Understanding the changes on both a macro and micro level is pivotal in further improving your health and what you can expect from growing up in regards to sleep. Furthermore, its been shown that sex does play a role in determining your level of sleep disruption as you age. With this evidence its clear to see that how you sleep is how you age.
Citations
Adam M, Rétey JV, Khatami R, Landolt HP. Age-related changes in the time course of vigilant attention during 40 hours without sleep in men. Sleep. 2006;29:55–57. [PubMed] [Google Scholar]
Andrade KC, Spoormaker VI, Dresler M, Wehrle R, Holsboer F, Sämann PG, Czisch M. Sleep spindles and hippocampal functional connectivity in human NREM sleep. J Neurosci. 2011;31:10331–10339. [PMC free article] [PubMed] [Google Scholar]
Antonenko D, Diekelmann S, Olsen C, Born J, Mölle M. Napping to renew learning capacity: enhanced encoding after stimulation of sleep slow oscillations. Eur J Neurosci. 2013;37:1142–1151. [PubMed] [Google Scholar]
Backhaus J, Born J, Hoeckesfeld R, Fokuhl S, Hohagen F, Junghanns K. Midlife decline in declarative memory consolidation is correlated with a decline in slow wave sleep. Learn Mem. 2007;14:336–341. [PMC free article] [PubMed] [Google Scholar]
Backhaus W, Kempe S, Hummel FC. The effect of sleep on motor learning in the aging and stroke population - a systematic review. Restor Neurol Neurosci. 2015;34:153–164. [PubMed] [Google Scholar]
Baran B, Mantua J, Spencer RM. Age-related changes in the sleep-dependent reorganization of declarative memories. J Cogn Neurosci. 2016;28:792–802. [PMC free article] [PubMed] [Google Scholar]
Barrett-Connor E, Dam TT, Stone K, Harrison SL, Redline S, Orwoll E Osteoporotic Fractures in Men Study Group. The association of testosterone levels with overall sleep quality, sleep architecture, and sleep-disordered breathing. J Clin Endocrinol Metab. 2008;93:2602–2609. [PMC free article] [PubMed] [Google Scholar]
Beenhakker MP, Huguenard JR. Neurons that fire together also conspire together: is normal sleep circuitry hijacked to generate epilepsy? Neuron. 2009;62:612–632. [PMC free article] [PubMed] [Google Scholar]
Bergmann TO, Mölle M, Diedrichs J, Born J, Siebner HR. Sleep spindle-related reactivation of category-specific cortical regions after learning face-scene associations. Neuroimage. 2012;59:2733–2742. [PubMed] [Google Scholar]
Borbély AA. A two process model of sleep regulation. Hum Neurobiol. 1982;1:195–204. [PubMed] [Google Scholar]
Bouras C, Hof PR, Giannakopoulos P, Michel JP, Morrison JH. Regional distribution of neurofibrillary tangles and senile plaques in the cerebral cortex of elderly patients: a quantitative evaluation of a one-year autopsy population from a geriatric hospital. Cereb Cortex. 1994;4:138–150. [PubMed] [Google Scholar]
Braak H, Del Tredici K. Potential pathways of abnormal tau and α-synuclein dissemination in sporadic Alzheimer’s and Parkinson’s diseases. Cold Spring Harb Perspect Biol. 2016;8:8. [PMC free article] [PubMed] [Google Scholar]
Braak H, Thal DR, Ghebremedhin E, Del Tredici K. Stages of the pathologic process in Alzheimer disease: age categories from 1 to 100 years. J Neuropathol Exp Neurol. 2011;70:960–969. [PubMed] [Google Scholar]
Brayet P, Petit D, Frauscher B, Gagnon JF, Gosselin N, Gagnon K, Rouleau I, Montplaisir J. Quantitative EEG of rapid-eye-movement sleep: A marker of amnestic mild cognitive impairment. Clin EEG Neurosci. 2015;47:134–141. [PubMed] [Google Scholar]
Brownell SE, Conti B. Age- and gender-specific changes of hypocretin immunopositive neurons in C57Bl/6 mice. Neurosci Lett. 2010;472:29–32. [PMC free article] [PubMed] [Google Scholar]
Burke DM, Light LL. Memory and aging: the role of retrieval processes. Psychol Bull. 1981;90:513–514. [PubMed] [Google Scholar]
Carrier J, Viens I, Poirier G, Robillard R, Lafortune M, Vandewalle G, Martin N, Barakat M, Paquet J, Filipini D. Sleep slow wave changes during the middle years of life. Eur J Neurosci. 2011;33:758–766. [PubMed] [Google Scholar]
Cavuoto MG, Ong B, Pike KE, Nicholas CL, Bei B, Kinsella GJ. Objective but not subjective sleep predicts memory in community-dwelling older adults. J Sleep Res. 2016;25:475–485. [PubMed] [Google Scholar]
Chee MW, Choo WC. Functional imaging of working memory after 24 hr of total sleep deprivation. J Neurosci. 2004;24:4560–4567. [PMC free article] [PubMed] [Google Scholar]
Chee CA, Roozendaal B, Swaab DF, Goudsmit E, Mirmiran M. Vasoactive intestinal polypeptide neuron changes in the senile rat suprachiasmatic nucleus. Neurobiol Aging. 1988;9:307–312. [PubMed] [Google Scholar]
Cheng JT, Liu IM, Juang SW, Jou SB. Decrease of adenosine A-1 receptor gene expression in cerebral cortex of aged rats. Neurosci Lett. 2000;283:227–229. [PubMed] [Google Scholar]
Cirelli C, Huber R, Gopalakrishnan A, Southard TL, Tononi G. Locus ceruleus control of slow-wave homeostasis. J Neurosci. 2005;25:4503–4511. [PMC free article] [PubMed] [Google Scholar]
Clewett DV, Lee TH, Greening S, Ponzio A, Margalit E, Mather M. Neuromelanin marks the spot: identifying a locus coeruleus biomarker of cognitive reserve in healthy aging. Neurobiol Aging. 2016;37:117–126. [PMC free article] [PubMed] [Google Scholar]
Conte F, Arzilli C, Errico BM, Giganti F, Iovino D, Ficca G. Sleep measures expressing ‘functional uncertainty’ in elderlies’ sleep. Gerontology. 2014;60:448–457. [PubMed] [Google Scholar]
Craik FI. Age differences in human memory. In: Birren JE, Schaie KW, editors. Handbook of the Psychology of Aging. New York: Van Nostrand Reinhold; 1977. pp. 384–420. [Google Scholar]
De Gennaro L, Ferrara M. Sleep spindles: an overview. Sleep Med Rev. 2003;7:423–440. [PubMed] [Google Scholar]
de Sarro GB, Bagetta G, Ascioti C, Libri V, Nisticó G. Micro-infusion of clonidine and yohimbine into locus coeruleus alters EEG power spectrum: effects of aging and reversal by phosphatidylserine. Br J Pharmacol. 1988;95:1278–1286. [PMC free article] [PubMed] [Google Scholar]
de Souza L, Smaili SS, Ureshino RP, Sinigaglia-Coimbra R, Andersen ML, Lopes GS, Tufik S. Effect of chronic sleep restriction and aging on calcium signaling and apoptosis in the hippocampus of young and aged animals. Prog Neuropsychopharmacol Biol Psychiatry. 2012;39:23–30. [PubMed] [Google Scholar]
Delacourte A, Sergeant N, Wattez A, Maurage CA, Lebert F, Pasquier F, David JP. Tau aggregation in the hippocampal formation: an ageing or a pathological process? Exp Gerontol. 2002;37:1291–1296. [PubMed] [Google Scholar]
Diekelmann S, Born J. The memory function of sleep. Nat Rev Neurosci. 2010;11:114–126. [PubMed] [Google Scholar]
Dijk DJ, Beersma DG, van den Hoofdakker RH. All night spectral analysis of EEG sleep in young adult and middle-aged male subjects. Neurobiol Aging. 1989;10:677–682. [PubMed] [Google Scholar]
Dijk DJ, Duffy JF, Riel E, Shanahan TL, Czeisler CA. Ageing and the circadian and homeostatic regulation of human sleep during forced desynchrony of rest, melatonin and temperature rhythms. J Physiol. 1999;516:611–627. [PMC free article] [PubMed] [Google Scholar]
Dijk DJ, Groeger JA, Stanley N, Deacon S. Age-related reduction in daytime sleep propensity and nocturnal slow wave sleep. Sleep. 2010;33:211–223. [PMC free article] [PubMed] [Google Scholar]
Downs JL, Dunn MR, Borok E, Shanabrough M, Horvath TL, Kohama SG, Urbanski HF. Orexin neuronal changes in the locus coeruleus of the aging rhesus macaque. Neurobiol Aging. 2007;28:1286–1295. [PubMed] [Google Scholar]
Dubé J, Lafortune M, Bedetti C, Bouchard M, Gagnon JF, Doyon J, Evans AC, Lina JM, Carrier J. Cortical thinning explains changes in sleep slow waves during adulthood. J Neurosci. 2015;35:7795–7807. [PMC free article] [PubMed] [Google Scholar]
Dumay N. Sleep not just protects memories against forgetting, it also makes them more accessible. Cortex. 2016;74:289–296. [PubMed] [Google Scholar]
Dworak M, McCarley RW, Kim T, Kalinchuk AV, Basheer R. Sleep and brain energy levels: ATP changes during sleep. J Neurosci. 2010;30:9007–9016. [PMC free article] [PubMed] [Google Scholar]
Eggert T, Dorn H, Sauter C, Nitsche MA, Bajbouj M, Danker-Hopfe H. No effects of slow oscillatory transcranial direct current stimulation (tDCS) on sleep-dependent memory consolidation in healthy elderly subjects. Brain Stimulat. 2013;6:938–945. [PubMed] [Google Scholar]
Ehlers CL, Kupfer DJ. Slow-wave sleep: do young adult men and women age differently? J Sleep Res. 1997;6:211–215. [PubMed] [Google Scholar]
Ekonomou A, Pagonopoulou O, Angelatou F. Age-dependent changes in adenosine A1 receptor and uptake site binding in the mouse brain: an autoradiographic study. J Neurosci Res. 2000;60:257–265. [PubMed] [Google Scholar]
Feinberg I, Carlson VR. Sleep variables as a function of age in man. Arch Gen Psychiatry. 1968;18:239–250. [Google Scholar]
Feld GB, Wilhelm I, Ma Y, Groch S, Binkofski F, Mölle M, Born J. Slow wave sleep induced by GABA agonist tiagabine fails to benefit memory consolidation. Sleep. 2013;36:1317–1326. [PMC free article] [PubMed] [Google Scholar]
Fell J, Klaver P, Lehnertz K, Grunwald T, Schaller C, Elger CE, Fernández G. Human memory formation is accompanied by rhinal-hippocampal coupling and decoupling. Nat Neurosci. 2001;4:1259–1264. [PubMed] [Google Scholar]
Fogel SM, Albouy G, Vien C, Popovicci R, King BR, Hoge R, Jbabdi S, Benali H, Karni A, Maquet P, et al. fMRI and sleep correlates of the age-related impairment in motor memory consolidation. Hum Brain Mapp. 2013;35:3625–3645. [PMC free article] [PubMed] [Google Scholar]
Fogel S, Vien C, Karni A, Benali H, Carrier J, Doyon J. Sleep spindles: A physiological marker of age-related changes in grey matter in brain regions supporting motor skill memory consolidation. Neurobiol Aging. 2016;49:154–164. [PubMed] [Google Scholar]
Foley DJ, Vitiello MV, Bliwise DL, Ancoli-Israel S, Monjan AA, Walsh JK. Frequent napping is associated with excessive daytime sleepiness, depression, pain, and nocturia in older adults: findings from the National Sleep Foundation ‘2003 Sleep in America’ Poll. Am J Geriatr Psychiatry. 2007;15:344–350. [PubMed] [Google Scholar]
Gagnon JF, Petit D, Latreille V, Montplaisir J. Neurobiology of sleep disturbances in neurodegenerative disorders. Curr Pharm Des. 2008;14:3430–3445. [PubMed] [Google Scholar]
Garcia-Falgueras A, Ligtenberg L, Kruijver FP, Swaab DF. Galanin neurons in the intermediate nucleus (InM) of the human hypothalamus in relation to sex, age, and gender identity. J Comp Neurol. 2011;519:3061–3084. [PubMed] [Google Scholar]
Gorgoni M, Lauri G, Truglia I, Cordone S, Sarasso S, Scarpelli S, Mangiaruga A, D’Atri A, Tempesta D, Ferrara M, et al. Parietal fast sleep spindle density decrease in Alzheimer’s disease and amnesic mild cognitive impairment. Neural Plast. 2016;2016:8376108. [PMC free article] [PubMed] [Google Scholar]
Guzman-Marin R, Bashir T, Suntsova N, Szymusiak R, McGinty D. Hippocampal neurogenesis is reduced by sleep fragmentation in the adult rat. Neuroscience. 2007;148:325–333. [PMC free article] [PubMed] [Google Scholar]
Halassa MM, Florian C, Fellin T, Munoz JR, Lee SY, Abel T, Haydon PG, Frank MG. Astrocytic modulation of sleep homeostasis and cognitive consequences of sleep loss. Neuron. 2009;61:213–219. [PMC free article] [PubMed] [Google Scholar]
Hall-Porter JM, Schweitzer PK, Eisenstein RD, Ahmed HA, Walsh JK. The effect of two benzodiazepine receptor agonist hypnotics on sleep-dependent memory consolidation. J Clin Sleep Med. 2014;10:27–34. [PMC free article] [PubMed] [Google Scholar]
Harman SM, Metter EJ, Tobin JD, Pearson J, Blackman MR Baltimore Longitudinal Study of Aging. Longitudinal effects of aging on serum total and free testosterone levels in healthy men. J Clin Endocrinol Metab. 2001;86:724–731. [PubMed] [Google Scholar]
Harrison Y, Horne JA, Rothwell A. Prefrontal neuropsychological effects of sleep deprivation in young adults–a model for healthy aging? Sleep. 2000;23:1067–1073. [PubMed] [Google Scholar]
Hassainia F, Petit D, Nielsen T, Gauthier S, Montplaisir J. Quantitative EEG and statistical mapping of wakefulness and REM sleep in the evaluation of mild to moderate Alzheimer’s disease. Eur Neurol. 1997;37:219–224. [PubMed] [Google Scholar]
Hita-Yañez E, Atienza M, Gil-Neciga E, Cantero JL. Disturbed sleep patterns in elders with mild cognitive impairment: the role of memory decline and ApoE ε4 genotype. Curr Alzheimer Res. 2012;9:290–297. [PubMed] [Google Scholar]
Holth JK, Patel TK, Holtzman DM. Sleep in Alzheimer’s disease–beyond amyloid. Neurobiol Sleep Circadian Rhythms. 2016;2:4–14. [PMC free article] [PubMed] [Google Scholar]
Hunt NJ, Rodriguez ML, Waters KA, Machaalani R. Changes in orexin (hypocretin) neuronal expression with normal aging in the human hypothalamus. Neurobiol Aging. 2015;36:292–300. [PubMed] [Google Scholar]
Ingiosi AM, Opp MR, Krueger JM. Sleep and immune function: glial contributions and consequences of aging. Curr Opin Neurobiol. 2013;23:806–811. [PMC free article] [PubMed] [Google Scholar]
Irwin MR. Why sleep is important for health: a psychoneuroimmunology perspective. Annu Rev Psychol. 2015;66:143–172. [PMC free article] [PubMed] [Google Scholar]
Ishunina TA, van Heerikhuize JJ, Ravid R, Swaab DF. Estrogen receptors and metabolic activity in the human tuberomamillary nucleus: changes in relation to sex, aging and Alzheimer’s disease. Brain Res. 2003;988:84–96. [PubMed] [Google Scholar]
Jack CR, Jr, Knopman DS, Jagust WJ, Shaw LM, Aisen PS, Weiner MW, Petersen RC, Trojanowski JQ. Hypothetical model of dynamic biomarkers of the Alzheimer’s pathological cascade. Lancet Neurol. 2010;9:119–128. [PMC free article] [PubMed] [Google Scholar]
Kakimoto A, Ito S, Okada H, Nishizawa S, Minoshima S, Ouchi Y. Age-related sex-specific changes in brain metabolism and morphology. J Nucl Med. 2016;57:221–225. [PubMed] [Google Scholar]
Kales A, Wilson T, Kales JD, Jacobson A, Paulson MJ, Kollar E, Walter RD. Measurements of all-night sleep in normal elderly persons: effects of aging. J Am Geriatr Soc. 1967;15:405–414. [PubMed] [Google Scholar]
Kang JE, Lim MM, Bateman RJ, Lee JJ, Smyth LP, Cirrito JR, Fujiki N, Nishino S, Holtzman DM. Amyloid-beta dynamics are regulated by orexin and the sleep-wake cycle. Science. 2009;326:1005–1007. [PMC free article] [PubMed] [Google Scholar]
Kasanuki K, Iseki E, Kondo D, Fujishiro H, Minegishi M, Sato K, Katsuse O, Hino H, Kosaka K, Arai H. Neuropathological investigation of hypocretin expression in brains of dementia with Lewy bodies. Neurosci Lett. 2014;569:68–73. [PubMed] [Google Scholar]
Kessler BA, Stanley EM, Frederick-Duus D, Fadel J. Age-related loss of orexin/hypocretin neurons. Neuroscience. 2011;178:82–88. [PMC free article] [PubMed] [Google Scholar]
King BR, Saucier P, Albouy G, Fogel SM, Rumpf JJ, Klann J, Buccino G, Binkofski F, Classen J, Karni A, Doyon J. Cerebral activation during initial motor learning forecasts subsequent sleep-facilitated memory consolidation in older adults. Cereb Cortex. 2016:bhv347. [PubMed] [Google Scholar]
Klerman EB, Dijk DJ. Age-related reduction in the maximal capacity for sleep–implications for insomnia. Curr Biol. 2008;18:1118–1123. [PMC free article] [PubMed] [Google Scholar]
Klöppel S, Kovacs GG, Voigtlander T, Wanschitz J, Flicker H, Hainfellner JA, Guentchev M, Budka H. Serotonergic nuclei of the raphe are not affected in human ageing. Neuroreport. 2001;12:669–671. [PubMed] [Google Scholar]
Ladenbauer J, Külzow N, Passmann S, Antonenko D, Grittner U, Tamm S, Flöel A. Brain stimulation during an afternoon nap boosts slow oscillatory activity and memory consolidation in older adults. Neuroimage. 2016;142:311–323. [PubMed] [Google Scholar]
Landolt HP, Borbély AA. Age-dependent changes in sleep EEG topography. Clin Neurophysiol. 2001;112:369–377. [PubMed] [Google Scholar]
Landolt HP, Dijk DJ, Achermann P, Borbély AA. Effect of age on the sleep EEG: slow-wave activity and spindle frequency activity in young and middle-aged men. Brain Res. 1996;738:205–212. [PubMed] [Google Scholar]
Latta F, Leproult R, Tasali E, Hofmann E, L’Hermite-Balériaux M, Copinschi G, Van Cauter E. Sex differences in nocturnal growth hormone and prolactin secretion in healthy older adults: relationships with sleep EEG variables. Sleep. 2005;28:1519–1524. [PubMed] [Google Scholar]
Liguori C, Romigi A, Nuccetelli M, Zannino S, Sancesario G, Martorana A, Albanese M, Mercuri NB, Izzi F, Bernardini S, et al. Orexinergic system dysregulation, sleep impairment, and cognitive decline in Alzheimer disease. JAMA Neurol. 2014;71:1498–1505. [PubMed] [Google Scholar]
Lim AS, Kowgier M, Yu L, Buchman AS, Bennett DA. Sleep fragmentation and the risk of incident Alzheimer’s disease and cognitive decline in older persons. Sleep. 2013a;36:1027–1032. [PMC free article] [PubMed] [Google Scholar]
Lim AS, Yu L, Kowgier M, Schneider JA, Buchman AS, Bennett DA. Modification of the relationship of the apolipoprotein E ε4 allele to the risk of Alzheimer disease and neurofibrillary tangle density by sleep. JAMA Neurol. 2013b;70:1544–1551. [PMC free article] [PubMed] [Google Scholar]
Lim AS, Ellison BA, Wang JL, Yu L, Schneider JA, Buchman AS, Bennett DA, Saper CB. Sleep is related to neuron numbers in the ventrolateral preoptic/intermediate nucleus in older adults with and without Alzheimer’s disease. Brain. 2014;137:2847–2861. [PMC free article] [PubMed] [Google Scholar]
Lim AS, Fleischman DA, Dawe RJ, Yu L, Arfanakis K, Buchman AS, Bennett DA. Regional neocortical gray matter structure and sleep fragmentation in older adults. Sleep. 2016;39:227–235. [PMC free article] [PubMed] [Google Scholar]
Lo JC, Sim SK, Chee MW. Sleep reduces false memory in healthy older adults. Sleep. 2014;37:665–671. 671A. [PMC free article] [PubMed] [Google Scholar]
Lo JC, Groeger JA, Cheng GH, Dijk DJ, Chee MW. Self-reported sleep duration and cognitive performance in older adults: a systematic review and meta-analysis. Sleep Med. 2016;17:87–98. [PubMed] [Google Scholar]
Lord C, Sekerovic Z, Carrier J. Sleep regulation and sex hormones exposure in men and women across adulthood. Pathol Biol (Paris) 2014;62:302–310. [PubMed] [Google Scholar]
Luppi PH, Peyron C, Fort P. Not a single but multiple populations of GABAergic neurons control sleep. Sleep Med Rev. 2016 Published online March 12, 2016
Mackiewicz M, Nikonova EV, Zimmermann JE, Romer MA, Cater J, Galante RJ, Pack AI. Age-related changes in adenosine metabolic enzymes in sleep/wake regulatory areas of the brain. Neurobiol Aging. 2006;27:351–360. [PubMed] [Google Scholar]
Mander BA, Santhanam S, Saletin JM, Walker MP. Wake deterioration and sleep restoration of human learning. Curr Biol. 2011;21:R183–R184. [PMC free article] [PubMed] [Google Scholar]
Mander BA, Rao V, Lu B, Saletin JM, Lindquist JR, Ancoli-Israel S, Jagust W, Walker MP. Prefrontal atrophy, disrupted NREM slow waves and impaired hippocampal-dependent memory in aging. Nat Neurosci. 2013;16:357–364. [PMC free article] [PubMed] [Google Scholar]
Mander BA, Rao V, Lu B, Saletin JM, Ancoli-Israel S, Jagust WJ, Walker MP. Impaired prefrontal sleep spindle regulation of hippocampal-dependent learning in older adults. Cereb Cortex. 2014;24:3301–3309. [PMC free article] [PubMed] [Google Scholar]
Mander BA, Marks SM, Vogel JW, Rao V, Lu B, Saletin JM, Ancoli-Israel S, Jagust WJ, Walker MP. β-amyloid disrupts human NREM slow waves and related hippocampus-dependent memory consolidation. Nat Neurosci. 2015;18:1051–1057. [PMC free article] [PubMed] [Google Scholar]
Mander BA, Winer JR, Jagust WJ, Walker MP. Sleep: A novel mechanistic pathway, biomarker, and treatment target in the pathology of Alzheimer’s disease? Trends Neurosci. 2016a;39:552–566. [PMC free article] [PubMed] [Google Scholar]
Mander BA, Zhu A, Lindquist JR, Villeneuve S, Rao V, Lu B, Saletin JM, Ancoli-Israel S, Jagust W, Walker MP. Degeneration of white matter pathways in older adults explains the failure of sleep spindles to promote motor memory consolidation. Sleep. 2016b;39:A22. [Google Scholar]
Marshall L, Helgadóttir H, Mölle M, Born J. Boosting slow oscillations during sleep potentiates memory. Nature. 2006;444:610–613. [PubMed] [Google Scholar]
Martin N, Lafortune M, Godbout J, Barakat M, Robillard R, Poirier G, Bastien C, Carrier J. Topography of age-related changes in sleep spindles. Neurobiol Aging. 2013;34:468–476. [PubMed] [Google Scholar]
Mary A, Schreiner S, Peigneux P. Accelerated long-term forgetting in aging and intra-sleep awakenings. Front Psychol. 2013;4:750. [PMC free article] [PubMed] [Google Scholar]
Mednick SC, McDevitt EA, Walsh JK, Wamsley E, Paulus M, Kanady JC, Drummond SP. The critical role of sleep spindles in hippocampal-dependent memory: a pharmacology study. J Neurosci. 2013;33:4494–4504. [PMC free article] [PubMed] [Google Scholar]
Menkes-Caspi N, Yamin HG, Kellner V, Spires-Jones TL, Cohen D, Stern EA. Pathological tau disrupts ongoing network activity. Neuron. 2015;85:959–966. [PubMed] [Google Scholar]
Meyer PT, Elmenhorst D, Boy C, Winz O, Matusch A, Zilles K, Bauer A. Effect of aging on cerebral A1 adenosine receptors: A [18F] CPFPX PET study in humans. Neurobiol Aging. 2007;28:1914–1924. [PubMed] [Google Scholar]
Miller SL, Celone K, DePeau K, Diamond E, Dickerson BC, Rentz D, Pihlajamäki M, Sperling RA. Age-related memory impairment associated with loss of parietal deactivation but preserved hippocampal activation. Proc Natl Acad Sci USA. 2008;105:2181–2186. [PMC free article] [PubMed] [Google Scholar]
Monk TH. Aging human circadian rhythms: conventional wisdom may not always be right. J Biol Rhythms. 2005;20:366–374. [PubMed] [Google Scholar]
dos Moraes WS, Poyares DR, Guilleminault C, Ramos LR, Bertolucci PH, Tufik S. The effect of donepezil on sleep and REM sleep EEG in patients with Alzheimer disease: a double-blind placebo-controlled study. Sleep. 2006;29:199–205. [PubMed] [Google Scholar]
Münch M, Knoblauch V, Blatter K, Schröder C, Schnitzler C, Kräuchi K, Wirz-Justice A, Cajochen C. The frontal predominance in human EEG delta activity after sleep loss decreases with age. Eur J Neurosci. 2004;20:1402–1410. [PubMed] [Google Scholar]
Latest Longevity Research Straight to your Inbox
Sign up for The Longevity Blueprint, a weekly newsletter from Healthspan analyzing the latest longevity research.
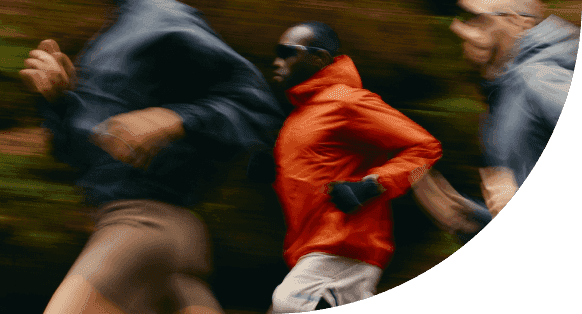
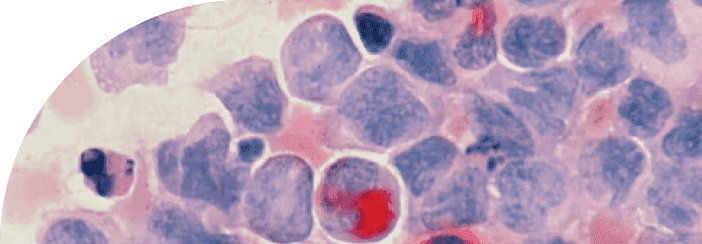

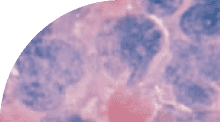