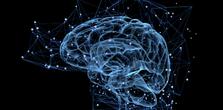
Understanding the Aging Brain: The Role of Biomarkers in Predicting Neurological Health
As the human brain ages, it undergoes extensive structural and functional transformations that predispose individuals to neurodegenerative diseases. Recognizing these changes early is pivotal, as it could lead to timely intervention strategies. Current research is thus increasingly directed towards identifying reliable biomarkers that can predict the pace and nature of brain aging. These biomarkers are essential for crafting targeted interventions that could delay or alleviate the symptoms of age-related neurological conditions. This review consolidates recent progress in identifying such biomarkers and their efficacy in prognosticating alterations in brain health. We delve into the physiological changes characteristic of an aging brain and discuss state-of-the-art techniques for their quantification. Furthermore, we provide a critical analysis of the present research landscape while also projecting future trends. Our comprehensive review aims to shed light on how these emergent biomarkers could change our approach to managing neurological health in the elderly.
20 mins
By: Shreshtha Jolly, Shriya Bakhshi
Introduction
As we age, our brains undergo a range of structural and functional changes, increasing our vulnerability to neurodegenerative diseases. In response to this challenge, researchers are intensely focused on identifying biomarkers that can accurately predict the rate and nature of brain aging. Such biomarkers are crucial for developing targeted preventive and therapeutic strategies aimed at delaying or mitigating the onset of age-related neurological disorders.
This article reviews recent advancements in the field, exploring how these biomarkers can effectively forecast changes in brain health. We will examine the physiological transformations that occur as the brain ages, discussing the latest research on methods for measuring these changes. Our analysis will cover both the current landscape and future directions in research on brain aging, providing a comprehensive overview of how emerging biomarkers could potentially transform our approach to neurological health in later life.
Structural and Physiological Changes in the Aging Brain
Brain Volume Changes
Like a grand estate weathering over time, our brain undergoes significant transformations as we age. One of the most notable changes is the reduction in brain volume and weight. This shrinkage is particularly pronounced in the frontal lobe and hippocampus—regions crucial for memory and cognitive functions. [1]
Researchers have identified detailed patterns of brain volume changes across the lifespan through a comprehensive meta-analysis that included 56 longitudinal studies. This analysis showed that brain volume grows at an annual rate of 1% until the age of nine, then transitions from growth to shrinkage around thirteen. In early adulthood, the volume remains relatively stable, then begins to shrink by about 0.2% annually starting at age thirty-five, accelerating to a decrease of 0.5% per year from the age of sixty onwards. [2]
Understanding the implications of this shrinkage requires a grasp of several key concepts [3]:
Gray Matter: This is where the cell bodies of neurons reside, functioning as the brain's "computing center." It is involved in processing information for functions such as sight, hearing, memory, emotions, and decision-making.
White Matter: Comprising nerve fibers sheathed in myelin, white matter acts like the brain's wiring, facilitating communication between different brain areas and aiding in the rapid transmission of messages.
Cortex: The thin outer layer of gray matter, the cortex is involved in complex functions like thinking, planning, and processing sensory information.
Sulci: These minor grooves on the cortical surface not only give the brain its characteristic wrinkled appearance but also increase its surface area, allowing more gray matter to fit within the confines of the skull.
Ventricles: Fluid-filled spaces within the brain, ventricles help cushion the brain against injury, assist in waste removal, and regulate internal pressure.
Age-related reductions in brain volume manifest in various forms: loss of white and gray matter tissues, cortical thinning, sulcal widening, and ventricular enlargement. These changes, intensifying particularly after the age of fifty, have been well documented across multiple studies. [4] [5]
Blood Flow Changes
As we age, not only does the structure of our brain change, but there is also a significant reduction in blood flow to the brain. This diminished flow restricts the delivery of essential oxygen and nutrients to brain cells, compromising their functional capacities. The scenario is akin to a dwindling river that no longer adequately nourishes the surrounding land. [1]
One of the primary reasons for reduced blood flow is the loss of elasticity in blood vessels due to aging. Over time, the blood vessels in our brain can become stiffer and more prone to damage. This stiffening is often exacerbated by cerebral atherosclerosis (CA), a condition in which the arterial walls thicken and harden, becoming less flexible. Additionally, excessive collagen may accumulate in the vessel walls as we age, narrowing the channels through which blood can flow and further reducing the supply of critical resources to the brain’s white matter. [6]
These vascular changes can impair the brain's ability to eliminate waste products via the bloodstream, leading to permanent damage to the white matter. Such damage can decrease the density of blood vessels in the affected regions, further complicating blood flow regulation and exacerbating the deterioration. This cyclical process highlights the critical link between vascular health and brain function, underscoring the importance of maintaining cardiovascular health as a means of protecting cognitive abilities in older age. [7]
Neurotransmitter Alterations
A significant aspect of brain aging involves changes in neurotransmitter synthesis and release. Neurotransmitters are chemical messengers that facilitate communication between neurons (brain cells). To visualize their function, imagine neurotransmitters as river channels carrying packets (signals) from one port (the sending neuron) to another (the receiving neuron).
In the brain, a diverse array of neurotransmitters plays specific roles in regulating behavior and cognitive functions. Typically, neurotransmitters are released from what are called presynaptic neurons into a gap known as the synapse, which is the space between adjacent neurons. Once in the synapse, neurotransmitters bind to specific receptors on the postsynaptic neuron, initiating various physiological responses. [8]
For example, dopamine, a neurotransmitter, is associated with feelings of pleasure, satisfaction, and motivation upon binding to its receptors. However, as we age, the production of dopamine, the levels of its receptors, and its binding efficiency all decrease. Research indicates that dopamine levels decline by about 10% per decade starting from early adulthood. This decline is linked to reductions in cognitive and motor functions, as dopamine also supports these processes. [9]
Similarly, serotonin, another critical neurotransmitter, is essential for promoting feelings of well-being, relaxation, and confidence. Deficiencies in serotonin can lead to anxiety, depression, sleep disturbances, and an increased craving for carbohydrates. Regrettably, serotonin levels also decrease with age, contributing to common age-related challenges such as depression and sleep issues. [10][11][12]
Understanding Biomarkers in Brain Aging
To appreciate how biomarkers can predict the onset of brain aging, it is crucial to first define what biomarkers are. Biomarkers are measurable indicators of biological states or conditions within an organism. These can be genes, proteins, molecules, or specific characteristics of cells and tissues. Commonly, biomarkers are detected in bodily fluids such as blood, urine, and saliva, or directly in bodily tissues. The presence, absence, or levels of these markers can reveal information about both normal and pathological processes occurring in the body.
When it comes to brain aging, biomarkers are invaluable tools for assessing the aging process within the brain. They provide tangible data that can reveal the actual age, structural integrity, and functional capacity of the brain. This article will focus on two primary types of biomarkers for brain aging: functional biomarkers, which assess the operational aspects of the brain, and body fluid biomarkers, which are typically measured in fluids like cerebrospinal fluid (CSF) and blood. Each category offers unique insights into how the brain changes with age.
Assessing Brain Functions Through Functional Markers
The brain performs various functions including cognitive processes, motor coordination, sensory perception, and emotional regulation. Each of these functions is affected differently by aging. Functional markers, which indicate the level of brain functionality, can help assess the impact of aging on these functions.
Cognitive Function: Cognition involves how our brains process and utilize information to learn and solve problems, encompassing memory, language, understanding, and decision-making. As people age, overall cognitive function typically declines. Episodic memory, a type of long-term memory that involves recollection of specific events and experiences, is particularly sensitive to aging. Research has shown that episodic memory declines with age, associated with atrophy in brain regions such as the temporal lobe, posterior cingulate gyrus, and hippocampus, along with reduced white matter in older adults. The decline in episodic memory can be measured using cognitive tests like the Cued Recall Test and the Auditory Verbal Learning Test (AVLT). These tests assess a person's ability to recall information after delays and recognize previously presented information, providing insights into their episodic memory capabilities. [13][14][15][16]
Motor Coordination: Motor skills, which involve the ability to perform movements and actions effectively, also decline with age. This decline has been correlated with reductions in gray matter volume in studies involving large cohorts. Motor function can be evaluated through tests like the Spiral Drawing Test, which assesses hand-eye coordination and precision, and the Small Pegboard Test, which measures dexterity and finger control. These assessments help in understanding how aging affects motor skills and overall brain structure. [18][19]
Standard Lab Markers
As part of a comprehensive approach to understanding the aging brain, standard laboratory markers provide crucial insights into various physiological aspects that influence neurological health. These biomarkers help in the early detection, monitoring, and management of age-related changes and conditions.
RBC Magnesium
Magnesium in red blood cells (RBCs) offers a reliable indicator of long-term magnesium status in the body. Unlike serum magnesium levels, which can fluctuate and only reflect immediate magnesium status, RBC magnesium levels provide a more consistent and comprehensive overview, capturing magnesium content inside the cells where it performs most of its critical functions.
Adequate magnesium levels are particularly important for brain health. Magnesium contributes to the maintenance of the nervous system's structural integrity and optimizes its functionality. It influences various neurotransmitter systems, including those that regulate the release of dopamine, serotonin, and gamma-aminobutyric acid (GABA), all of which are vital for mood regulation and cognitive functions.
Research has demonstrated that magnesium promotes synaptic plasticity, the ability of synapses (the connections between neurons) to strengthen or weaken over time. This plasticity is crucial for learning and memory formation. Magnesium acts at the synaptic level by influencing the NMDA (N-methyl-D-aspartate) receptors, which are involved in the synaptic plasticity underlying learning and memory. Studies have shown that an increase in brain magnesium levels can lead to the enhancement of learning abilities, working memory, and short- and long-term memory in both young and aged animals.
Additionally, adequate magnesium levels can help protect the brain from various stressors, including neurotoxic damage from heavy metals and excitotoxicity from overactive glutamate receptors. It also plays a role in protecting against inflammation and oxidative stress within the brain, which can exacerbate the aging process and contribute to the development of neurodegenerative diseases such as Alzheimer's disease. [26]
hs-CRP (High-sensitivity C-reactive Protein)
High-sensitivity C-reactive protein (hs-CRP) is a sensitive marker used to evaluate low levels of inflammation in the body. Produced by the liver in response to inflammation, CRP levels increase when there's inflammation anywhere in the body. Measuring hs-CRP provides valuable insights particularly in cases where chronic, low-grade inflammation is suspected. While it is widely recognized for its role in cardiovascular health, indicating inflammation in the cardiovascular system that can lead to heart disease, its relevance extends significantly into neurology.
Chronic inflammation is a critical factor in the development and progression of neurodegenerative diseases, including Alzheimer’s disease and Parkinson’s disease. In the brain, persistent inflammation can lead to the activation of microglia, the brain’s primary immune cells. When chronically activated, these cells can produce inflammatory cytokines and reactive oxygen species that may contribute to neuronal damage and the characteristic neurodegeneration seen in these conditions.
Studies have shown that elevated hs-CRP levels are associated with an increased risk of developing conditions like Alzheimer’s disease. Higher hs-CRP levels have been correlated with greater cognitive decline and an increased rate of the hippocampal atrophy often observed in Alzheimer’s patients. Additionally, elevated hs-CRP has been linked to other inflammatory neurological conditions, such as multiple sclerosis and ischemic stroke, indicating its broad applicability in neurology. [27]
It's important to remember that hs-CRP is a nonspecific marker of inflammation. It can be elevated due to a variety of reasons unrelated to neurological conditions. Thus, while it's a useful marker, it should be interpreted within a broader clinical context.
Vitamin D
Vitamin D plays a significant role in brain health and neurological function. This nutrient facilitates the absorption of calcium and phosphorus, which are crucial for maintaining healthy communication between the brain and other parts of the body. Additionally, vitamin D is involved in numerous brain processes, making it vital for cognitive functioning and mental health.Vitamin D receptors are especially prevalent in areas of the brain associated with complex planning, processing of information, and the formation of new memories.
Research has consistently linked insufficient levels of vitamin D to an increased risk of cognitive decline and neurodegenerative diseases. Low levels of this vitamin have been associated with a higher risk of developing Alzheimer's disease, Parkinson's disease, and multiple sclerosis. Studies have shown that individuals with lower levels of vitamin D experience cognitive decline at a faster rate compared to those with adequate levels, which can manifest as difficulties in thinking, problem-solving, and remembering.
Moreover, vitamin D has neuroprotective effects. It helps regulate critical neurotransmitters like dopamine and serotonin, influencing mood and behavior. Vitamin D also plays a role in neuroplasticity, which is crucial for learning new information and adapting to new experiences. The neuroprotective properties of vitamin D include its anti-inflammatory and antioxidant actions that can mitigate the effects of aging on the brain, potentially reducing the risk of dementia and other cognitive impairments. [28]
Liver Function Biomarkers
The liver's role extends beyond just processing nutrients and detoxifying harmful substances; it is crucial for overall brain health. This key organ metabolizes toxins and drugs, preventing potentially harmful substances from reaching the brain, where they could cause damage or disrupt normal brain function. Additionally, the liver synthesizes several essential proteins and enzymes that influence brain metabolism and the regulation of neurotransmitters, which are critical for cognitive functions. Commonly tested liver function markers include alanine aminotransferase (ALT), aspartate aminotransferase (AST), alkaline phosphatase (ALP), bilirubin, and albumin levels.
Liver disease can have a direct and detrimental effect on cognitive function, a condition often referred to as hepatic encephalopathy. This occurs when the liver cannot effectively remove toxins from the blood due to impairment or disease, leading to toxin accumulation in the bloodstream. These toxins can cross the blood-brain barrier and directly affect brain function.
Furthermore, the liver plays a significant role in regulating blood glucose levels, which is crucial for maintaining the energy supply to the brain. Fluctuations in glucose levels can affect brain function, impacting cognitive abilities and mood. Consistent liver function ensures stable glucose levels, thereby supporting uninterrupted brain activity and neural stability. [29]
Homocysteine
Homocysteine is an amino acid produced by the body, usually as a byproduct of consuming meat. While it is a normal part of the metabolism, elevated levels of homocysteine in the blood have been linked to an increased risk of several serious health issues, particularly in the brain.
Elevated homocysteine levels can cause endothelial damage, which is damage to the inner lining of blood vessels. This damage contributes to the development of atherosclerosis, a condition where the arteries become clogged and hardened, restricting blood flow. In the brain, reduced blood flow can lead to vascular complications that may not only increase the risk of stroke but also contribute to the development of vascular dementia.
Additionally, high homocysteine levels have been associated with an increased risk of developing Alzheimer’s disease. Research suggests that excessive homocysteine may promote the production of amyloid-beta peptides and tau phosphorylation, which are hallmarks of Alzheimer’s pathology. It may also induce oxidative stress and inflammation, further damaging neurons and impairing cognitive function. [30]
The biomarkers listed here can be measured through routine lab work. They serve as key tools for a holistic understanding of factors affecting brain health. By regularly monitoring these markers, individuals can implement preventive and therapeutic strategies that enhance cognitive function and overall neurological well-being. This proactive approach can help mitigate the risk of developing serious neurological conditions and improve the quality of life as individuals age.
Advanced Lab Markers
Several substances in body fluids, beyond those measured in routine labs, are gaining recognition for their potential to predict brain health. These markers can reflect various aspects of neuronal health and activity.
Tau
Tau protein is primarily located in the axons of neurons, which are crucial for transmitting electrical signals throughout the nervous system. Axons are long, slender projections that extend from the neuron's cell body, facilitating the communication between neurons or between neurons and other types of cells. Many axons are covered by a myelin sheath, which speeds up signal transmission. At the end of axons are terminals that release neurotransmitters, bridging the communication gap at synapses with other cells.
In certain conditions, the tau protein, which plays a key role in maintaining the structure and function of these axons, can become chemically altered. This alteration leads to the formation of tau aggregates, which are clumps of this protein that accumulate abnormally. Elevated levels of these tau aggregates in the cerebrospinal fluid (CSF) and blood are used as biomarkers for neurodegenerative diseases because they indicate ongoing damage and dysfunction within brain neurons. [21]
Research has demonstrated that elevated levels of tau in the blood are associated with memory impairments and cognitive decline in aging populations. A notable longitudinal study involving 276 healthy adults found that increased levels of plasma tau were correlated with cerebral atrophy and could predict susceptibility to Alzheimer's Disease. [22]
The concept of atrophy refers to the reduction in size, function, or number of cells in an organ or tissue. In the context of brain health, cerebral atrophy means the loss of neurons and the connections between them, leading to a decrease in brain volume. This condition is often observed in neurodegenerative diseases such as Alzheimer's Disease, where elevated tau levels and their aggregation are linked to the progressive decline in brain function. [22]
This connection underscores the importance of tau as a biomarker, as it helps in predicting the susceptibility to conditions like Alzheimer’s Disease, providing insights into the structural integrity and functional capacity of the brain during aging and disease progression.
Neurofilament Light Chain (NfL)
NfL, a protein integral to the structural framework of neurons, can be detected in body fluids like blood and cerebrospinal fluid (CSF). Research has demonstrated its potential as a predictive biomarker in the context of brain aging and neurodegenerative diseases.
A notable study by Kaiser et al. (2021) analyzed plasma levels of NfL across a diverse age range, including 122 participants aged 21 to 107 years. The findings were particularly significant among centenarians (individuals over 100 years old), where plasma NfL levels were better predictors of mortality than traditional morbidity scales, which assess brain and physical functioning. [23]
Parallel findings in animal research reinforce the predictive value of NfL. Studies involving mice have shown that plasma NfL levels increase with age. Interestingly, dietary restriction regimens known to extend lifespan in mice were associated with reduced age-related increases in serum NfL levels. These results highlight the utility of NfL as a biomarker for aging and age-related pathologies, offering insights that could guide interventions to mitigate the impacts of aging on the brain. [23]
Glial fibrillary acidic protein (GFAP)
Glial Fibrillary Acidic Protein (GFAP) is a protein found in body fluids that has significant implications for diagnosing and predicting Alzheimer's Disease (AD). To fully understand its role, we first need to consider the biological processes involved in AD. One of the primary features of Alzheimer’s is the formation of amyloid plaques in the brain. These plaques are mainly composed of a protein called beta-amyloid (Aβ), which originates from a larger molecule, amyloid-beta precursor protein (APP). APP is embedded in the membrane of neural cells and can be processed through two pathways: the non-amyloidogenic pathway, which is the normal, healthy pathway where APP is cleaved by an enzyme called α-secretase into harmless fragments, and the amyloidogenic pathway, which leads to the formation of Aβ. In the amyloidogenic pathway, APP is first cleaved by an enzyme known as β-APP-cleaving enzyme-1 (BACE1), resulting in a fragment called C99. C99 is further processed to release Aβ, which aggregates to form plaques contributing to neurotoxic damage and the progression of Alzheimer's Disease. [24]
Research, including a study by Shir et al. (2022), has investigated how GFAP levels relate to Alzheimer's pathology. This study involved brain scans to visualize Aβ burden and blood tests to measure plasma GFAP levels in 200 participants. The findings indicated that higher levels of GFAP were linked to the presence of Aβ plaques, decreased cortical thickness, and microbleeds in the brain, even after adjusting for variables such as age and sex. However, once the presence of amyloid was accounted for, the link between GFAP and another protein involved in AD, tau, was not significant. This suggests that GFAP is particularly sensitive to changes related to amyloid plaque presence rather than other aspects of Alzheimer's pathology. [25]
These findings underscore the value of GFAP as a biomarker in blood for detecting and monitoring Alzheimer’s Disease. By reflecting the extent of amyloid plaque buildup and associated brain changes, GFAP helps in the early diagnosis and could potentially guide therapeutic strategies to mitigate the impacts of this devastating disease. In essence, GFAP provides a window into the brain’s pathological state, offering crucial insights that could improve the management of Alzheimer's in clinical settings. [25]
These advanced biomarkers found in body fluids such as blood plasma, urine, and cerebrospinal fluid (CSF) are crucial for studying brain aging due to their non-invasive collection, high sensitivity, and accurate measurement capabilities. Tau, NfL (Neurofilament Light Chain), and GFAP (Glial Fibrillary Acidic Protein), have each shown promise in predicting brain health and age-related pathologies. However, despite their potential, measuring these biomarkers presents several challenges:
Sensitivity and Specificity: Accurately detecting and quantifying these biomarkers requires highly sensitive and specific analytical techniques. Small variations in technique or sample handling can significantly affect the results.
Biological Variability: Levels of biomarkers can vary significantly between individuals due to genetic differences, lifestyle, and even the time of day when samples are collected.
Technological Limitations: Some biomarkers, especially those present in very low concentrations in blood or urine, require advanced detection technologies that may not be widely available in all clinical settings.
Interpretation Challenges: The presence of a biomarker does not always correlate directly with disease severity or progression, complicating their use in clinical diagnostics and monitoring.
Though several challenges currently exist in measuring advanced body fluid biomarkers for brain aging, scientific advancements are paving the way for easier and more reliable methods. Developments in bioanalytical methods, such as more sensitive mass spectrometry techniques and advanced immunoassays, are improving our ability to detect low-abundance biomarkers in body fluids like blood and urine. These technologies reduce the need for large sample volumes and enhance the accuracy and reliability of biomarker measurements.
These advancements are making it increasingly feasible to use advanced biomarkers in routine clinical practice and research, improving our understanding of brain aging and potentially leading to earlier detection and better management of age-related neurological conditions.
Conclusion
The study of biomarkers relevant to the aging brain is an evolving field that holds promise for transforming our approach to neurodegenerative diseases. Through meticulous research and the ongoing development of technologies, we are beginning to understand how changes in brain volume, blood flow, and neurotransmitter levels contribute to the cognitive decline associated with aging. This deeper insight allows for the potential to not only predict but also mitigate the progression of age-related neurological disorders.
While the technology for advanced biomarkers continues to evolve, it's important to recognize the value of the tools already at our disposal. Standard laboratory markers and functional assessments provide a robust foundation for assessing brain health. These readily available tools can effectively monitor changes in critical areas such as magnesium levels, inflammatory markers like hs-CRP, vitamin D status, liver function, and homocysteine levels, all of which have direct implications for neurological health.
By integrating these existing methods with emerging biomarkers, we enhance our ability to offer tailored preventive measures and therapeutic strategies. This comprehensive approach, which combines established techniques with cutting-edge research, enriches our understanding and management of the aging brain. It underscores our capacity to not just react to age-related changes but to anticipate and address them proactively, improving the quality of life for the aging population and offering significant advances in our fight against neurodegenerative diseases.
TAKE HOME POINTS
Brain Volume and Structural Changes: The brain experiences a reduction in volume and weight as we age, particularly in the frontal lobe and hippocampus, which are crucial for memory and cognitive functions. As we age, our brain experiences a reduction in both white and gray matter, which are crucial for processing and transmitting information. Additionally, the brain's outer layer thins, the grooves on its surface widen, and the fluid-filled spaces inside it enlarge, all of which can impact brain function.
Blood Flow Changes: Aging leads to reduced blood flow to the brain due to the loss of elasticity in blood vessels and conditions like cerebral atherosclerosis. These vascular changes can impair the brain's ability to eliminate waste products and contribute to white matter damage.
Neurotransmitter Alterations: Aging affects neurotransmitter levels and functions, notably those of dopamine and serotonin, which are crucial for mood regulation and cognitive functions. The decrease in neurotransmitters contributes to common age-related challenges such as depression and cognitive decline.
Combatting Brain Aging: Engaging in regular physical exercise, following a diet rich in omega-3 fatty acids and antioxidants, staying mentally active through puzzles and new skills, and managing stress effectively are key strategies that can help preserve cognitive functions and delay the effects of aging on the brain.
Biomarkers are measurable indicators of biological states or conditions and are crucial for assessing the aging process within the brain. They provide insights into the brain’s actual age, structural integrity, and functional capacity. RBC Magnesium: This test measures magnesium levels inside red blood cells to assess how much magnesium is available in your body over the long term. Magnesium is crucial for brain functions like memory and learning because it helps nerve cells communicate effectively.
hs-CRP (High-sensitivity C-reactive Protein): This is a test for a protein that your body produces in response to inflammation. Since inflammation can contribute to brain aging and diseases like Alzheimer's, monitoring hs-CRP levels can help gauge inflammation-related risks to brain health.
Vitamin D: Vitamin D is important for brain health because it helps maintain nerve and brain function. Low levels of vitamin D have been linked to a higher risk of problems like memory loss and other brain disorders.
Liver Function Biomarkers: These tests check the health of your liver, which helps detoxify harmful substances and manage nutrients that affect brain health. Poor liver function can lead to cognitive problems and affect overall brain function.
Homocysteine: Homocysteine is an amino acid in your blood that, at high levels, can damage blood vessels and lead to brain disorders like stroke and dementia. Keeping homocysteine levels in check is important for maintaining good blood flow to the brain. Tau: Tau is a protein that helps stabilize internal structures of nerve cells. When tau proteins malfunction and clump together, they can disrupt brain function, which is commonly seen in Alzheimer’s disease. Testing for tau can help detect these changes early on.
Neurofilament Light Chain (NfL): This marker is found in nerve cells, and high levels in the blood or spinal fluid can indicate that nerve cells are damaged. This is useful for detecting diseases that cause loss of brain cells, like multiple sclerosis or Alzheimer's.
Glial Fibrillary Acidic Protein (GFAP): GFAP is a protein involved in the brain’s response to injury and disease. Elevated levels can indicate brain damage or inflammation, often seen in conditions like Alzheimer's disease. Monitoring GFAP can help understand and track these changes.
Citations
Latest Longevity Research Straight to your Inbox
Sign up for The Longevity Blueprint, a weekly newsletter from Healthspan analyzing the latest longevity research.
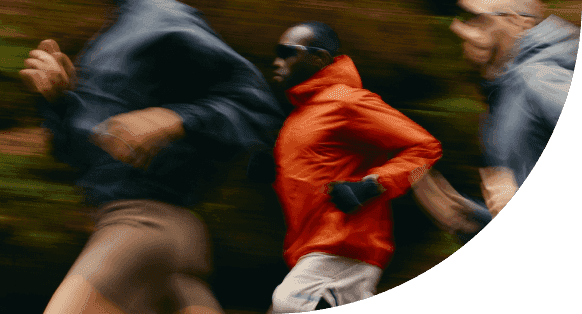
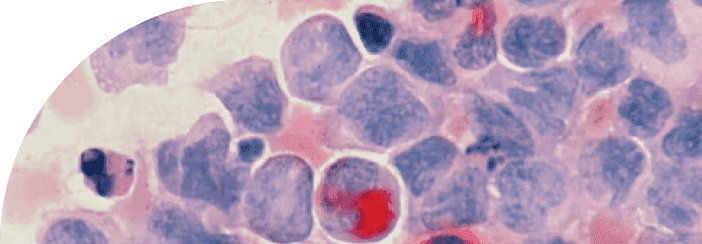

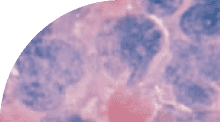