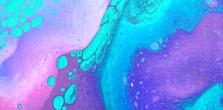
Exploring the Antagonistic Hallmarks of Aging and Potential Interventions
In the second part of our Hallmarks of Aging series, we explore the "Antagonistic Hallmarks," which include Cellular Senescence, Mitochondrial Dysfunction, Deregulated Nutrient Sensing, Dysbiosis, Chronic Inflammation, Altered Cellular Communication, and Stem Cell Exhaustion. We uncover how these processes intricately shape our aging trajectory. Additionally, we'll explore interventions that have shown promise in reducing the harm caused by these hallmarks, paving the way for a better understanding of potential strategies to slow down the aging process.
Cellular Senescence
rapamycin
24 mins
By: Jacob Rose
In the first article of this two-part series, you were introduced to the hallmarks of aging, twelve highly integrated aspects of the aging process. Exacerbating these hallmarks increases a person’s rate of aging, and ameliorating them will slow aging.
We covered a few ways to interact with the “primary” drivers of aging that begin at the smallest building blocks of our cellular machinery. In this article, we will cover the remaining 7 hallmarks of aging, beginning with the “antagonistic” hallmarks.
All of the hallmarks are integrated and intertwine with one another, but these, in particular, are considered antagonistic because they largely result from the primary stress of the previous hallmarks like genomic instability or loss of proteostasis, and exacerbate aging in response. Cellular senescence is the perfect segue into this type of hallmark because, at its core, senescence is a necessary stress response.
Cellular Senescence
In the early days of cellular biology, it was believed that human cells were “immortal”; careful scientists could expand a single group of cells for infinite divisions as long as they didn’t forget to feed them or accidentally contaminate the dish. It wasn’t until Leonard Hayflick discovered that there is a limit to cellular division, now coined the “Hayflick Limit”. Thus, the first instance of cellular senescence was recorded.
Decades have since passed, and the field has significantly advanced, expanding beyond Leonard Hayflick's initial discovery of "replicative senescence." This concept is primarily linked to the reduction in telomere length. As cells approach their "Hayflick Limit" — a term referring to the maximum number of times a cell population can divide before it halts — the progressively shortening telomeres serve as an indicator of deteriorating cell health. Ultimately, the diminished telomeres trigger a series of reactions that irreversibly impede cell division.
Cellular senescence evolved to prevent genomically unstable or possibly cancerous cells from proliferating. However, one of the major reasons this protective mechanism is featured as a hallmark of aging is the way senescent cells ask your immune system to come clean them up.
The defining characteristic of a senescent cell is the inflammatory distress signal that it emits. Because a senescent cell is in a damaged state, it signals out to other cells that it is under duress by emitting a witch's brew of inflammatory molecules that we call the SASP.
The Senescence Associated Secretory Phenotype, more commonly known as SASP, is a complex blend of molecules that senescent cells produce. Think of SASP as a biological distress signal, which is intended to attract the local immune system. Throughout our lives, these senescent cells and their SOS-like SASP signals are managed effectively, keeping a balance within our cellular environment.
However, as we advance in age, an increasing number of our cells have shortened telomeres—protective caps at the end of our DNA strands that decrease with each cell division—or experience significant stress that pushes them into a senescent state.
These conditions lead to an accumulation of senescent cells and an increase in SASP signaling, which can overwhelm the immune system's ability to respond. This inability to effectively manage the increased cellular "noise" is a key component of the aging process.
This age-associated accumulation of senescent cells and their SASP contribute to systemic aging and intensify the pro-inflammatory phenotype observed in aging. Luckily, there is a ton of research occurring in this particular field and multiple drugs, some already considered safe to administer, are being tested in the clinic for diseases with strong ties to senescent cells.
Senolytics and Senomorphics
Senolytics are drugs designed to selectively kill senescent cells, whereas senomorphics are drugs designed to limit the detrimental effects of the SASP. Senolytics designed to selectively kill senescent cells have had limited clinical success but show continual promise in model organisms. This fact alone suggests that the role of senescent cells is complicated and their removal may not be a perfect answer in all cases.
It is also important to realize that senescent cells make up a relatively small population of the cells in our body. This is of particular interest because senescent cells beget “secondary” senescent cells through the SASP. This process generates small, dense clusters of susceptible cells that senesce with enough time.
Some accounts have senescent cells occupying only 1% of cells in our body; other reports suggest this population is closer to 20% in certain organ systems. Regardless of their true proportion in our bodies, their pro-inflammatory nature and increasing prevalence in age-related diseases make them fantastic targets for anti-aging therapies.
Rapamycin and Cellular Senescence
Rapamycin is the most well researched intervention to target cellular senescence. Rapamycin inhibits a protein known as mammalian target of rapamycin (mTOR), which plays a central role in cell growth, proliferation, and survival.
In the context of aging, inhibiting mTOR with rapamycin has been shown to extend lifespan in yeast, worms, flies, and mice. Research suggests that it may do this by enhancing cellular "housekeeping" processes like autophagy, where cells recycle their own components, thereby reducing damage and dysfunction of senescent cells.
Additionally, studies have shown that rapamycin can suppress the detrimental effects of senescent cells. It does this by potentially altering the SASP, making senescent cells less inflammatory and harmful to their neighboring cells. Rapamycin may also boost immune system function, enabling the body to clear away senescent cells more effectively.
Interestingly, since Hayflick discovered replicative senescence, other stimuli have been found to induce senescence, including mitochondrial dysfunction, our next hallmark of aging.
Mitochondrial Dysfunction
The mitochondria may be the powerhouse of the cell, but there is more about their biology than what that phrase may suggest. It is unsurprising that the major energy-producing organelles in our cells are the cause of an incredibly complex hallmark of aging.
There is an abundance of mitochondria in our bodies. Each cell type differs in the number of mitochondria in each cell, but in general, the classroom graphic depicting a single mitochondria per cell is quite misleading. In fact, our cells are rife with these little energy producing pseudo-life forms.
There is a fascinating theory of cellular evolution where two separate, ancient cells come together in a symbiotic relationship to form eukaryotic ancestors. One is a bacteria-like organism that simplified itself to become the larger cell’s powerhouse.
Evidence for this is apparent in mitochondrial biology. Mitochondria have their own DNA, garbage systems, proteins, and enough energy production for themselves as well as the larger cell. With all of that in mind, there are a diverse group of problems that commonly arise and mitochondrial dysfunction is an umbrella term for the myriad processes being dysregulated with age.
Mitochondria produce a molecule used for energy called ATP. ATP production is a tightly regulated system that occurs by shuttling ions through the Electron Transport Chain (ETC), ending with ATP Synthase generating one of these ATP molecules.
One of the byproducts of this process is reactive oxygen species (ROS) which have been known for a long time to be toxic and generally bad for remaining young.
Reactive Oxygen Species (ROS)
Studies that remove ROS using antioxidants have shown context-dependent, transient benefits from doing so, but even though we understand the huge detriment of ROS, it seems that simply removing them will not solve all our age-related energetic problems.
Mitophagy
Another angle is to steal tenets of good health from another hallmark. I mentioned above that mitochondria have all the essential functions of basic life, and as such, they undergo a specialized version of autophagy called “mitophagy.”
Naturally, because there are many mitochondria in a cell, the less efficient ones will need to be identified and recycled. Normal mitochondria can keep ROS contained, but as mitochondrial membranes become leaky, the ROS escapes and upsets the natural electrical gradient that helps push ions around.
Mitochondria that cannot maintain this gradient need to be recycled just like other organelles. Supplements aimed at energetic wellness are plentiful, and knowing what we know about aging, likely have roles to fill on the individual level based on a person’s needs: lifestyle, exposures, genetics, etc.
The dream of totally personalized medicine is still in its infancy, and determining exactly what supplements do and how best to administer them is a daunting challenge. Another confounding factor for supplementation is how our cells detect nutrients and how they will modulate cellular function based on scarcity or surplus.
Deregulated Nutrient Sensing
Regulating the timing and intake of our nutrients is a remarkably tangible way to influence one of the primary hallmarks of aging. During the course of normal aging, absent of metabolic diseases, we possess significant control over our body's nutrient balance. This control allows us to directly influence our body's aging processes and potentially mitigate some age-related changes.
Our bodies typically perceive nutrients in a binary way: a state of growth or a state of starvation. This dichotomy sets off various protein cascades — complex sequences of biochemical reactions — that are largely influenced by growth factors and the mammalian target of rapamycin (mTOR). When abundant nutrients are sensed, these factors stimulate cell growth, proliferation, and differentiation, driving the body into a growth state.
Conversely, in the perceived absence of nutrients, the body shifts into a conservation mode, slowing down these processes. When deprived of nutrients, our cells become incredibly frugal with essential nutrients and metabolites.
Rapamycin and mTOR
One side effect of the starvation protocols is the subsequent activation of autophagy programs to keep cellular function as efficient as possible. This is why Rapamycin and other mTOR inhibitors are so attractive and efficacious, one compound will effectively trick our cells into thinking nutrients are scarce and trigger the downstream benefits like improved autophagy and dampen unnecessary growth.
Manipulating these protocols to benefit our bodies is an important part of healthy aging and can dramatically reduce the risk of metabolic disease as we age. Equally as important, obesity and BMI are potent risk factors for nearly all age-related diseases, so watching our weight is crucial to aging gracefully.
Caloric Restriction and Intermittent Fasting
Caloric restriction has proven effective in slowing aging time and time again, both in models and in humans when they can stick to a strict diet. Something that hasn’t been as robustly tested yet, but is showing similar promise is intermittent fasting (IF).
We are coming to understand that it is possible to enhance the benefits of starvation without constant denial of food by simply restricting when we eat. Intermittent fasting can be done on a daily basis by abstaining most of the day and only eating within a strict time window; alternatively, it has also been shown that 24 or 48 hour fasts every few weeks will have similar benefits.
The specifics of these time restrictions are still largely understudied, especially in humans, but in general, they are much more forgiving than strict caloric restriction. The best diet is the one you can stick to, and hopefully, as you age, your metabolic profile will align with what lifestyle will maintain.
Dieting, while often challenging, is a viable change anyone can make to interact with their health and there is no shortage of methods to experiment with.
At this point in the series, it should be clear that context is always incredibly important, and a person’s individual traits will affect how certain therapies and lifestyles alter their aging.
As mentioned above, these were the antagonistic hallmarks of aging, leaving only the four integrative hallmarks left! The integrative hallmarks are the final set to be presented because they typically appear after our bodies have failed to resolve the above hallmarks in a meaningful way.
The cycle of damage and inefficient repair leads to chronically unresolved problems over a number of years and will result in the appearance of these integrative hallmarks of aging in more compartments of our body.
Dysbiosis
Dysbiosis is the imbalance of our body’s natural relationship with resident microbes, mostly in the gut. Most of the current work in this field has been to characterize the diverse microbes that make up the gut microbiome and the changes in population diversity as we age.
In general, the gut microbiome gets less diverse as we age leading to narrower benefits provided by our biota. These preliminary understandings have allowed scientists to test hypotheses concerning effects of major alterations in the gut microbiome and its effects on disease and aging.
Novel experiments mixing and matching microbiomes in various models of aging using fecal microbiota transplantations (FMT) garnered results pointing to the gut microbiome as a crucial mediator of our immune system as well as a source of vital metabolites that may not be as readily produced by our cells.
Some of these metabolites have been studied well enough to know how they fluctuate during aging and how that fluctuation may be linked to the decline of the originating microbe in our gut.
Ongoing clinical studies are replacing specific cultures in efforts to supplement essential molecules like fatty acids, and surprisingly, increase insulin sensitivity. The consequences of a narrow microbiota are still being interrogated, but luckily promoting gut health is only a trip to the grocery store away.
There are commercially available probiotic supplements available in bulk as well as other foods that promote healthy guts, like yogurt and kombucha. The most important time to promote your microbiome is after antibiotic treatments. In extreme cases, some patients may even receive a FMT from a healthy donor. Logistically, it is strange to think about, but it may be necessary to raze your microbiome to clear a robust infection.
In these cases, it is most efficient to transplant a culture of health promoting microbes, more mild antibiotic treatments may only need a few days of yogurt or kombucha to come back to a healthier state. One of the major concerns tied to unwelcome members of the microbiome is the inflammation associated with their presence, which, when left unchecked becomes Chronic Inflammation.
Acarbose and the Microbiome
The relationship between acarbose and the gut microbiome is complex, but some studies suggest that acarbose can alter the composition of the gut microbiome. As acarbose inhibits the breakdown of complex carbohydrates in the small intestine, more carbohydrates reach the large intestine where they can be fermented by the gut microbiota. This process results in the production of short-chain fatty acids (SCFAs) such as butyrate, propionate, and acetate, which have been linked to a variety of health benefits, including improved gut health, reduced inflammation, and improved insulin sensitivity.
Moreover, by changing the availability of nutrients in the gut, acarbose could potentially favor the growth of some types of bacteria over others, altering the composition of the microbiome. Some research suggests that changes induced by acarbose might be beneficial, promoting the growth of bacteria associated with better metabolic health. However, the specifics of these changes can depend on several factors, including the individual's existing gut microbiome composition, diet, and other lifestyle factors.
Chronic Inflammation
Inflammation is a localized immune response that becomes simultaneously more prevalent and less effective as we age. Inflammaging was termed to describe this systemic elevation of pro-inflammatory molecules like Interleukin 6 (IL6) around the body.
Changes in IL6 can be detected in plasma and are also evident in a shift in our T cell populations. T cells are key immune cells that undergo many changes in their biology as they are exposed to and remember antigens from pathogenic bacteria or viruses. Once a T cell has recorded and reported an antigen to the larger immune system it can become exhausted and needs to be recycled.
Exhausted T cells aren’t as alert as fresh T cells, so their immunosurveillance capabilities are drastically reduced, which is the first step in inefficient clearance of viruses or malignant (cancerous or senescent) cells.
Inflammaging has been implicated in an increasing number of age-related diseases, including neurodegenerative diseases and cancer. In this respect, chronic inflammation is a highly integrated hallmark of aging, either by directly exacerbating or being triggered by the other hallmarks.
One example is in the case of mitochondrial dysfunction, those leaky mitochondrial membranes that can’t hold their ROS also cannot contain their circular mitochondrial DNA. To our immune systems, these circular sections of DNA look exactly like bacterial DNA and will trigger an immune response. Additionally, once the immune response has been triggered, it is the duty of autophagy machinery to clean up the pro-inflammatory molecules. So, what can we do about something so important, but also so ingrained in our biology?
Immune health is incredibly tricky to target and currently available treatments typically have drastic side effects. Immunosuppressing compounds absolutely have their benefits and crucial use cases, but there are few general use preventative therapies available today. Luckily, these therapies are being tested, both in the clinic and in model organisms. One strategy lies in filtering a few pro-inflammatory molecules from our circulation and there are a few interesting techniques to accomplish this.
Plasmapheresis is an emerging technology designed to remove plasma, filter it, supplement it, and replace it. Interesting data has emerged showing that even simply diluting unfavorable plasma factors is enough to promote health. This treatment also ameliorates the penultimate hallmark of aging, altered cellular communication.
Joan Mannick's Research on Rapamycin and Immunosenescence
New findings from researcher Joan Mannick shed light on the potential of mTOR inhibitors in improving immune function and combating age-related decline. Mannick's study focused on rapamycin and its analog RAD001, an mTOR inhibitor, and its effects on immunosenescence, the age-related decline in immune function.
Immunosenescence poses a significant health challenge, as it leads to increased susceptibility to infections and reduced response to vaccines, including influenza vaccination.
The study built upon previous research showing that rapamycin, another mTOR inhibitor, extended the lifespan of mice and improved various aging-related conditions. In elderly mice, rapamycin treatment rejuvenated immune function, resulting in increased production of new lymphocytes, improved response to influenza vaccination, and even extended lifespan.
Mannick's study aimed to investigate whether RAD001 could replicate these positive effects in elderly humans. The results were promising. RAD001 treatment appeared to ameliorate the age-related decline in immunological function in elderly volunteers, as evidenced by an enhanced response to influenza vaccination.
It is believed that RAD001 induced changes in specific cell populations that persisted even after treatment discontinuation. Notably, RAD001 reduced the percentage of PD-1-positive CD4 and CD8 T cells, which accumulate with age and exhibit diminished responses to antigen stimulation. By reducing PD-1 expression, RAD001 may have improved immune function and enhanced T cell responses in the elderly.
Furthermore, RAD001 treatment broadened the serologic response to influenza vaccination, leading to increased antibody titers against different strains of the virus. The results of Mannick's research suggest that rapamycin seems to rejuvenate the immune system.
Altered Cellular Communication
Cells typically engage in a complex 'conversation' with each other through the secretion and reception of specific signaling molecules, which serve as triggers for various cellular processes. Cellular components - encompassing DNA, RNA, proteins, and metabolites - each have unique ways of being sensed and interpreted. These components not only offer contextual information to the cell from which they originated, but also to other cells receiving these signals. This intricate communication network enables cells to respond effectively to changes in their environment, ensuring the smooth functioning of biological systems.
Biological systems are equipped with mechanisms for both long-range communication, as seen in the circulating factors in the blood and the neural signals of the nervous system, as well as short-range communication, where cells directly interact with each other or secrete substances into their immediate surroundings.
We've previously discussed the pivotal role of circulating factors in systemic inflammation. With this in mind, it's not difficult to imagine the potential consequences when these tightly regulated long-range cellular communications start to malfunction. Disruptions in these biological communication networks can contribute to various health issues, underlining the importance of maintaining these intricate cellular conversations in a balanced and functional state.
Short-range communications are conducted through a cell's intimate interactions within its microenvironment. A signaling cell can release a molecule or a blend of molecules directly into a neighboring cell. This exchange occurs through a complex network of microscopic channels, or tunnels, connecting cells, known as tight junctions. This precise cellular dialogue allows cells to respond immediately to changes, ensuring the optimal functioning and adaptation of tissues and organs.
These junctions are also incredibly important for structural cell types to maintain their barrier functions. For example, it is well known that as we age, our blood-brain barrier becomes leaky, allowing a larger, more diverse set of molecules to enter the brain than when we are young.
Even though this field needs further study to completely understand how barrier function in the brain declines, it is important to recognize the role these tight junctions may play in neurodegenerative diseases.
The extracellular matrix (ECM) is another critical component that physically interacts with cell clusters. The ECM is the non-cellular component that cells inhabit; it serves as a mechanical scaffold that supports cellular functions in a unique way. Some cells require a softer, more flexible environment, while others need a more rigid structure for optimal functioning.
Given this, changes in the ECM with age can significantly impact cellular function. These alterations can contribute to various diseases, including cancer and osteoarthritis. This underscores the importance of the ECM not just as a cellular support system, but as a key player in maintaining health and preventing disease progression.
One of the most dramatic attempts to repair cellular ECM is in cartilage replacement therapies for patients with osteoarthritis. Our bones and cartilage are highly specific structures made up of mostly ECM and because of this, replacing the substance that degrades with age seems like an elegant fix to a huge problem to an aging population.
Outcomes for these treatments are a mixed bag and are generally seen as treating a symptom, not necessarily the problem because it is the cells themselves that alter their ECM in such a way that it does not function properly. Without addressing the cells, they will just deteriorate the next ECM they encounter. ECM dysfunction is also tied closely to injury, which is a nice place to introduce the final aging hallmark, stem cell exhaustion.
Stem Cell Exhaustion
Stem cells, an intriguing group of cells within our bodies, function much like a multi-purpose tool at the cellular level. Picture a scenario where an injury triggers an intense bout of cellular stress. To alleviate this stress, our cells must access rarely utilized parts of the genome, akin to dusting off an old book in a vast library. Once these genetic segments are unveiled, the cellular machinery is set in motion to decode the stored information, ultimately helping to mitigate damage and initiate repair processes.
In our youth, responding to stress at the cellular level isn't overly challenging, largely because our epigenetic profiles - the chemical modifications that influence gene expression - are not rigidly fixed. This flexibility allows for easy access to genes that can respond to stress. To provide some context, one primary hallmark of aging involves these very epigenetic modifications.
Over time, these modifications can favor the efficient expression of certain genes due to their frequent need, or conversely, they may downregulate others due to their lesser use. However, as we age, these epigenetic modifications become more entrenched, possibly restricting the flexibility of our genetic response to stress, and thereby influencing our aging process.
The issue becomes very apparent in the face of a novel stress. If our cells have layers of epigenetic modifications designed to make everyday life and dealing with everyday stressors easier, it will be very difficult to get to the rarely encoded sections of the genome. This phenomenon is called cell plasticity and is directly related to stem cells, which are, by definition, incredibly plastic cells of a certain origin looking to help alleviate stress where applicable.
Aging uniquely effects each type of stem cell differently and will require an entire article on its own to go over, but the major point is that all cells, even the highly differentiated, epigenetically dusty ones, can exhibit “stemness” or cell plasticity in the face of damage to ease the injury.
As we age the epigenetic dust piles up, and our stem cell stores get depleted, but the damage comes all the same and the response to the damage is not as robust or flexible. The man who is credited with discovering the cocktail of molecules to induce this stemness won a Nobel Prize for it and thus, the Yamanaka Factors became starting points to improve stem cell exhaustion in aging.
The Yamanaka Factors are four proteins that robustly activate specific genetic programs that effectively knock the dust off heavily transcribed genes. Normal cells in culture will have an epigenetic age, whereas the same cells treated with Yamanaka Factors will be equivalent to a newborn.
Depending on the dose and time of exposure, fully differentiated cells will lose their identity and increase their stemness until they are considered “Induced Pluripotent Stem Cells” or IPSC’s. This technology alone has opened up the ability to model disease in incredible ways and Dr. Yamanaka is well deserving of the prize for this discovery, but it is not perfectly applicable to human health as it stands.
Cells need their identity to perform functions and incredibly plastic cells seldom work together in a way that cohesively contributes to human life. Additionally, intense treatment with these transcription factors causes cancer risk to skyrocket, making its progression a near certainty. However, it is incredible to think that the real issue is simply with dose or specificity. Scientists can and are working on that, in fact, it has already been shown that cyclic activation of these factors will ameliorate major aging phenotypes in mice without dramatically increasing the risk of cancer.
Rapamycin and Stem Cells
mTOR regulates stem cell behavior, influencing their capacity for self-renewal and differentiation, both of which are critical for tissue maintenance and repair. In this context, rapamycin has been found to be particularly beneficial for countering stem cell dysfunction, a characteristic feature of aging.
Stem cells, which are responsible for regenerating and repairing tissue, can decline in function with age, reducing their capacity to rejuvenate tissues and organs. Age-related dysfunction of stem cells can lead to a decrease in the body's ability to repair damage and maintain tissue health.
Rapamycin acts by inhibiting the activity of mTOR, a pathway that when hyperactivated, has been linked to stem cell dysfunction during aging. In doing so, rapamycin has been shown to rejuvenate stem cell function, effectively enhancing their regenerative potential. For instance, studies have demonstrated that rapamycin can restore the differentiation and proliferation potential of muscle-derived stem cells in mouse models of accelerated aging, and reduce senescence, a state of irreversible cell cycle arrest.
Overall, the ability of rapamycin to modulate stem cell function and slow down the rate of cellular aging underscores its potential as an anti-aging intervention. As research continues in this area, rapamycin may pave the way for more comprehensive and effective treatments for age-related diseases and conditions.
Closing Thoughts
As this informative two-part article comes to a close, it is hoped that it has sparked a newfound curiosity about the fascinating subject of aging. The introductory portion of the first article highlighted how dedicated scientists devote their entire labs and lives to studying even a single hallmark of aging. It's thanks to their relentless pursuit of knowledge that society is gaining the capacity to meaningfully engage with these hallmarks.
While some of the hallmarks still require comprehensive studies before high-confidence interventions can be made, it's crucial to understand that interacting with one hallmark often influences another. This interconnected influence underscores both the complexity and power inherent in the hallmarks of aging.
The trajectory of aging is determined by an intricate interplay of countless factors, including those explored in this article. Thus, it is essential for each person to explore personalized anti-aging strategies that best fit their individual needs. The exciting journey of scientific discovery continues, and its influences on the future of aging are eagerly anticipated.
TAKE HOME POINTS
The Hallmarks of Aging are fantastic opportunities to personalize our own aging experience and possible mitigate individual risk factors.
The primary hallmarks of aging are considered initial stressors that initiate the antagonistic hallmarks. Integrated hallmarks are pervasive and become more prevalent as the initial damage and downstream effects of the damage accumulate.
Each person will have their own aging trajectory that is based on their risk factors, exposures, and lifestyles. Interacting with high-risk hallmarks in safe and efficient ways will help slow aging.
Citations
Latest Longevity Research Straight to your Inbox
Sign up for The Longevity Blueprint, a weekly newsletter from Healthspan analyzing the latest longevity research.
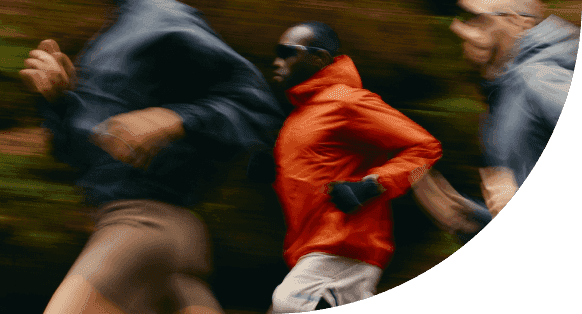
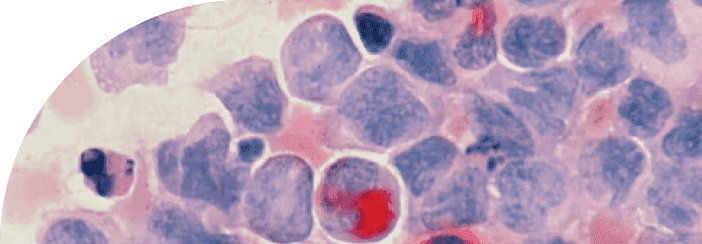

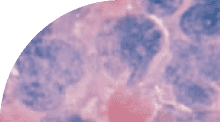